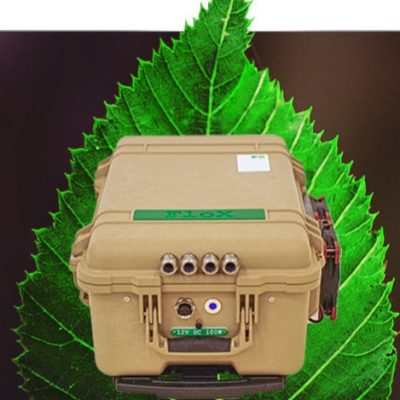
The FLOX Box is a self-contained dual hyperspectral system for monitoring long and short-term fluctuations in Solar-Induced Chlorophyll Fluorescence (SIF). SIF is considered the most direct, non-invasive remote-sensing signal for tracking photosynthetic activity and its dynamics at the leaf, canopy, ecosystem, and global scale. Accurate measurements of SIF are crucial for understanding photosynthesis and its dynamics in the field. SIF may be used to monitor changes in plant and algal responses to environmental factors that may affect growth and yield, including temperature, water and nutrient availability, the effects of herbicides and pesticides, pollution, herbivory, plant pathogens and other abiotic and biotic stresses. The FLOX represents the most advanced system available for SIF measurements in agriculture, forestry and other remote locations.
Qubit Systems has a long history of working with Photon Systems Instruments in providing technology for the active measurement of chlorophyll fluorescence on the laboratory scale (see: https://qubitsystems.com/products/plants-algae-soil/chlorophyll-fluorescence/) and in high-throughput phenotyping applications (read MORE). In addition, we now represent JB Hyperspectral of the GDR to provide the novel FLOX system with a dual spectrometer configuration for passive, long-term canopy scale measurements of SIF.
The SIF signal responds instantaneously to changes in environmental conditions such as light quantity and quality and can be monitored to track the effects of longer-term environmental factors that may cause stress, such as water deficits, nutrient deficiency, and disease states. Chlorophyll fluorescence constitutes represents only a small fraction (typically 2-5%) of the total light energy emitted (mostly as reflectance) from the leaf canopy, radiance at the top of the canopy, which is mostly composed of reflected sunlight, so monitoring requires a sophisticated device for high spectral resolution, sensitivity, and data processing.
The FLOX consists of two high-performance spectrometers contained in a temperature-controlled compartment. One fine resolution spectrometer (FLUO) covers the range from 650 nm to 800 nm observing the SIF signal within the O2A and O2B Fraunhofer Absorption lines. The second spectrometer (FULL) covers the range from 400 to 1000 nm. Each spectrometer measures the downwelling irradiance and the upwelling radiance using two optical fibres. A rugged weatherproof case and an autonomous acquisition protocol including data storage allows continuous observation of plant canopies over long periods.
All data are transferred automatically via LAN, WiFi or mobile internet to the secure JB Hyperspectral facility. Data are also recorded locally to an integrated SD card.
Clients have password-protected access to their remote data and can manipulate data via JB Hyperspectral’s intuitive user interface.
- Solar-Induced Chlorophyll Fluorescence Dynamics.
- Agriculture, forestry and horticulture.
- Effects of drought and temperature stress.
- Effects of nutrient regimes.
- Precision Agriculture.
- Effects of heavy metals and other pollutants.
- Monitoring effects of plant pathogens and herbivores.
- Long-term tracking of effects of climate change.
- Ground-truthing of satellite data.
OPTICAL
- Wavelength range: optic1 ~ 650–800 nm; optic2~ 400–950 mn
- Spectral Sampling Interval (SSI): optic1~ 0.17 nm; optic2 ~ 0.65 nm
- Spectral resolution (FWHM): optic1 ~ 0.3 nm; optic2 ~ 1.5 nm
- Signal to Noise Ratio (SNR): optic1 ~ 1000; optic2 ~ 250
- Field Of View (FOV): Dual FOV (Upwelling radiance ~25° Downwelling radiance 180°)
OPERATIONAL
- Signal Optimization: Automatic adaption to varying light conditions
- Dark current: Accurate dark current determination at each measurement cycle
- Manual acquisition: Interface software for manual measurement and calibration
- Automatic acquisition: Fully autonomous measurement mode for unattended data acquisition
- Quick measurements: 20 seconds under bright sunshine 60 seconds in overcast condition
- Stability: Reference system stability check and uncertainty estimates
- Simultaneous metadata: Spectrometer temperature, Outside temperature, GPS position, GPS time
- Data Display: Live assessment of the systems status
- Data storage: SD card up to 32 GB (12 months of measurements)
- Case: Robust and Waterproof housing based on the 1510 Pelicase
- Dimension: Small form factor (50 × 30 × 20 cm)
- Power supply: 12 Volt. From battery or solar panels
- Power consumption: Average consumption of 60 Watt. (20/100 Watt, cooling on/off)
- Energy saver: Day/night switch for energy saving
- Interfaces: RS232 via cable and wireless
OPTIONAL
- Dust Protection: Additional dust protection for Cosine Receptors
- Fiber Optics: Flexible length of fiber optics according to user needs
- Power supply: Solar panel and battery
- Communication: Add on for LAN/WLAN/Mobile Network Remote access
J. Zhu et al. (2023) The relationship between wheat yield and sun-induced chlorophyll fluorescence from continuous measurements over the growing season. Remote Sensing of Environment 2023. https://doi.org/10.1016/j.rse.2023.113791
V. Oehl, A. Damm (2023) WAFER: A new method to retrieve sun-induced fluorescence based on spectral wavelet decompositions. Remote Sensing of Environment 2023. https://doi.org/10.1016/j.rse.2023.113786
H. Tømmervik et al. (2023) The northernmost hyperspectral FLoX sensor dataset for monitoring of high-Arctic tundra vegetation phenology and Sun-Induced Fluorescence (SIF) https://doi.org/10.1016/j.dib.2023.109581
K. R Ahmed et al. (2023) Empirical insights on the use of sun-induced chlorophyll fluorescence to estimate short-term changes in crop transpiration under controlled water limitation. ISPRS Journal of Photogrammetry and Remote Sensing. 2023 https://doi.org/10.1016/j.isprsjprs.2023.07.016
B. Poulter et al. (2023) Simulating Global Dynamic Surface Reflectances for Imaging Spectroscopy Spaceborne Missions: LPJ-PROSAIL. Journal of Geophysical Research: Biogeosciences 2023. https://doi.org/10.1029/2022JG006935
T. Morozumi et al. (2023) Contributions of the understory and midstory to total canopy solar-induced chlorophyll fluorescence in a ground-based study in conjunction with seasonal gross primary productivity in a cool-temperate deciduous broadleaf forest. Remote sensing of Environment 2023. https://doi.org/10.1016/j.rse.2022.113340
C. van der Tol et al. (2023) Retrieval of chlorophyll fluorescence from a large distance using oxygen absorption bands. Remote Sensing of Environment 2023. https://doi.org/10.1016/j.rse.2022.113304
A. Burkart et al. (2022) Iterative design of a high light throughput cosine receptor fore optic for unattended proximal remote sensing. Journal of Applied Remote Sensing. 2022. https://doi.org/10.1117/1.JRS.16.044513
P. Naethe et al. (2022) A precise method unaffected by atmospheric reabsorption for ground-based retrieval of red and far-red sun-induced chlorophyll fluorescence. Agricultural and Forest Meteorology 2022. https://doi.org/10.1016/j.agrformet.2022.109152
R. Wang et al. (2022) Harmonizing solar induced fluorescence across spatial scales, instruments, and extraction methods using proximal and airborne remote sensing: A multi-scale study in a soybean field. Remote Sensing of Environment 2022. https://doi.org/10.1016/j.rse.2022.113268
R. Scodellaro et al. (2022) A novel hybrid machine learning phasor-based approach to retrieve a full set of solar induced fluorescence metrics and biophysical parameters. Remote Sensing of Environment https://doi.org/10.1016/j.rse.2022.113196
L. Wu et al. (2022) Physiological dynamics dominate the response of canopy far-red solar-induced fluorescence to herbicide treatment. Agricultural and Forest Meteorology 2022 https://doi.org/10.1016/j.agrformet.2022.109063
B. Buman et al. (2022) Towards consistent assessments of in situ radiometric measurements for the validation of fluorescence satellite missions. Remote Sensing of Environment 2022. https://doi.org/10.1016/j.rse.2022.112984
R. S.Gupana et al. (2022) Remote sensing of sun-induced chlorophyll-a fluorescence in inland and coastal waters: Current state and future prospects. Remote Sensing of Environment 2022. https://doi.org/10.1016/j.rse.2021.112482
A.Damm et al. (2022) Response times of remote sensing measured sun-induced chlorophyll fluorescence, surface temperature and vegetation indices to evolving soil water limitation in a crop canopy. Remote Sensing of Environment 2022 https://doi.org/10.1016/j.rse.2022.112957
Martini et al. (2021) Heatwave breaks down the linearity between sun-induced fluorescence and gross primary production. New Phytologist 2021 https://doi.org/10.1111/nph.17920
B. Dechant et al. (2022) NIRVP: A robust structural proxy for sun-induced chlorophyll fluorescence and photosynthesis across scales. Remote Sensing of Environment 2022 https://doi.org/10.1016/j.rse.2021.112763
S.De Cannière et al. (2021) Constraining water limitation of photosynthesis in a crop growth model with sun-induced chlorophyll fluorescence. Remote Sensing of Environment, 2021 https://doi.org/10.1016/j.rse.2021.112722
B. Siegmann et al. (2021) Downscaling of far-red solar-induced chlorophyll fluorescence of different crops from canopy to leaf level using a diurnal data set acquired by the airborne imaging spectrometer HyPlant. Remote sensing of environment 2021. https://doi.org/10.1016/j.rse.2021.112609
D. Hao et al. (2021) Practical approaches for normalizing directional solar-induced fluorescence to a standard viewing geometry. Remote Sensing of Environment, 2021 https://doi.org/10.1016/j.rse.2020.112171
Cochavi et al. (2021) Differential responses in a Mediterranean citrus orchard to two heatwave intensities is identified by combining measurements of fluorescence, carbonyl sulfide (COS), and CO2 uptake. New Phytologist 2021. https://doi.org/10.1111/nph.17247
A. Hornero et al. (2021) Assessing the contribution of understory sun-induced chlorophyll fluorescence through 3-D radiative transfer modelling and field data. Remote Sensing of Environment. 2021 https://doi.org/10.1016/j.rse.2020.112195
Choudhary K.K., Chakraborty A., Kumari M. (2021) Concepts and Applications of Chlorophyll Fluorescence: A Remote Sensing Perspective. Geospatial Technologies for Crops and Soils. Springer 2021. https://link.springer.com/chapter/10.1007/978-981-15-6864-0_7
Acebron et al. (2020) Diurnal dynamics of non-photochemical quenching in Arabidopsis npq mutants assessed by solar-induced fluorescence and reflectance measurements in the field. New Phytologist. 2020 https://doi.org/10.1111/nph.16984
Vilà-Guerau de Arellano et al. (2020) CloudRoots: integration of advanced instrumental techniques and process modelling of sub-hourly and sub-kilometre land–atmosphere interactions, Biogeosciences. 2020 https://doi.org/10.5194/bg-17-4375-2020
P. Yang et al. (2020) Fluorescence Correction Vegetation Index (FCVI): A physically based reflectance index to separate physiological and non-physiological information in far-red sun-induced chlorophyll fluorescence. Remote Sensing of Environment, 2020 https://doi.org/10.1016/j.rse.2020.111676
Z. Zhanga et al. (2020) Reduction of structural impacts and distinction of photosynthetic pathways in a global estimation of GPP from space-borne solar-induced chlorophyll fluorescence. Remote Sensing of Environment. 2020 https://doi.org/10.1016/j.rse.2020.111722
J. Quirós Vargas et al. (2020) Unmanned Aerial Systems (UAS)-Based Methods for Solar Induced Chlorophyll Fluorescence (SIF) Retrieval with Non-Imaging Spectrometers: State of the Art. Remote Sensing 2020 https://doi.org/10.3390/rs12101624
K. Biriukova et al. (2020) Effects of varying solar-view geometry and canopy structure on solar-induced chlorophyll fluorescence and PRI https://doi.org/10.1016/j.jag.2020.102069
G. H.Mohammed et al. (2019) Remote sensing of solar-induced chlorophyll fluorescence (SIF) in vegetation: 50 years of progress https://doi.org/10.1016/j.rse.2019.04.030
Petya K. E. et al. (2019) Diurnal and Seasonal Variations in Chlorophyll Fluorescence Associated with Photosynthesis at Leaf and Canopy Scales. Remote Sensing. 2019 https://doi.org/10.3390/rs11050488
G. Wohlfahrt et al. (2018) Sun-induced fluorescence and gross primary productivity during a heat wave. Scientific Reports. volume 8, Article number: 14169 https://www.nature.com/articles/s41598-018-32602-z
Sergio Cogliati, et al. (2019) A Spectral Fitting Algorithm to Retrieve the Fluorescence Spectrum from Canopy Radiance https://doi.org/10.3390/rs11161840
Javier Pacheco-Labrador et al. (2019) Sun-Induced Chlorophyll Fluorescence I: Instrumental Considerations for Proximal Spectroradiometers https://doi.org/10.3390/rs11080960
H. Aasen et al. (2019) Sun-Induced Chlorophyll Fluorescence II: Review of Passive Measurement Setups, Protocols, and Their Application at the Leaf to Canopy Level https://doi.org/10.3390/rs11080927
M. Pilar Cendrero-Mateo et al. (2019) Sun-Induced Chlorophyll Fluorescence III: Benchmarking Retrieval Methods and Sensor Characteristics for Proximal Sensing https://doi.org/10.3390/rs11080962
Julitta T. Wutzle et al. (2017) An R Package for Field Spectroscopy: From System Characterization to Sun-Induced Chlorophyll Fluorescence Retrieval. 17-19 January 2017, ESA ESRIN, Frascati (Rome), Italy. https://www.dropbox.com/s/w8umv5j9nvk5p5w/Abstract-book.pdf?dl=0
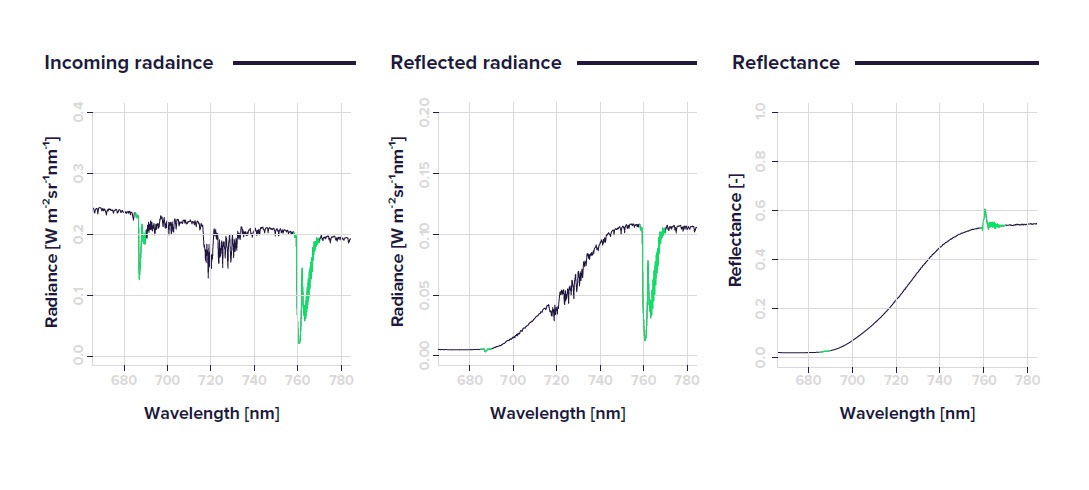